Hemodynamics of Leptomeningeal Collaterals after Large Vessel Occlusion and Blood Pressure Management with Endovascular Treatment
Article information
Abstract
Endovascular therapy (EVT) is an effective treatment for ischemic stroke due to large vessel occlusion (LVO). Unlike intravenous thrombolysis, EVT enables visualization of the restoration of blood flow, also known as successful reperfusion in real time. However, until successful reperfusion is achieved, the survival of the ischemic brain is mainly dependent on blood flow from the leptomeningeal collaterals (LMC). It plays a critical role in maintaining tissue perfusion after LVO via pre-existing channels between the arborizing pial small arteries or arterioles overlying the cerebral hemispheres. In the ischemic territory where the physiologic cerebral autoregulation is impaired and the pial arteries are maximally dilated within their capacity, the direction and amount of LMC perfusion rely on the systemic perfusion, which can be estimated by measuring blood pressure (BP). After the EVT procedure, treatment focuses on mitigating the risk of hemorrhagic transformation, potentially via BP reduction. Thus, BP management may be a key component of acute care for patients with LVO stroke. However, the guidelines on BP management during and after EVT are limited, mostly due to the scarcity of high-level evidence on this issue. In this review, we aim to summarize the anatomical and physiological characteristics of LMC to maintain cerebral perfusion after acute LVO, along with a landscape summary of the literature on BP management in endovascular treatment. The objective of this review is to describe the mechanistic association between systemic BP and collateral perfusion after LVO and thus provide clinical and research perspectives on this topic.
Introduction
The efficacy of intravenous thrombolysis (IVT) and endovascular therapy (EVT) for acute ischemic stroke patients after large vessel occlusion (LVO) has been established by large randomized clinical trials [1]. However, approximately 50% of patients still experience functional dependency despite successful recanalization after timely treatment [1]. Variability in collateral perfusion before recanalization may be a potential reason for this [2].
The fate of brain cells in the ischemic region beyond the LVO depends on perfusion through the leptomeningeal collateral (LMC) overlying the cerebral hemisphere. Inadequate LMCs, either due to anatomical paucity or physiological dysfunction, lead to rapid infarct growth and may contribute to the progression of ischemia after LVO [3]. LMCs are an evolutionarily conserved anatomic structure but show substantial variability by species and individuals [4]. Left ventricular contraction determines the degree of systemic blood circulation; similarly, the direction and flow of LMC perfusion depends on hydrostatic pressure gradients between the arteriolar and venular ends of cerebral blood vessels [5]. After the loss of cerebral autoregulation (CA) in the region of severely depressed perfusion [6], cerebral blood flow (CBF) to the ischemic territory is predominantly a function of systemic perfusion pressure. Thus, systemic blood pressure (BP) may be regarded as an indirect measure of LMC perfusion. Modulation of LMC perfusion via systemic BP could therefore help maximize functional benefits during acute stroke care.
This article outlines how knowledge of the anatomy and physiology of LMCs may be applied clinically and provides an overview of current evidence on BP management during and after endovascular treatment, as well as its influence on treatment outcomes. In the absence of randomized controlled trial evidence, the purpose of this review is to help stroke physicians develop an anatomo-physiological model-based approach to BP management while caring for patients with LVO stroke.
Anatomy and physiology of leptomeningeal collaterals
Anatomy
In a normal physiological state, blood flows from the heart to the brain via the anterior circulation, which comprises the internal carotid artery, the middle cerebral artery (MCA), which supplies the majority of this circulation, and the anterior cerebral artery (ACA). Blood also flows via the posterior circulation, which is formed by the vertebral artery, basilar artery, and posterior cerebral artery (PCA). The average diameter of the MCA at its origin is approximately 4 mm, roughly twice that of the ACA, which is approximately 2 mm [7,8]. As the amount of blood flow roughly corresponds to the vascular diameter, the average CBF in the MCA (146±31 mL/min) usually exceeds the flow in the ACA (82±18 mL/min) [9].
On the surface of the cerebral hemisphere, the pial arteries lie within the subarachnoid space and provide perforating arteries into the brain parenchyma. The proximal part of the perforating arteries usually consists of an endothelium surrounded by a thick basement membrane material with embedded smooth muscle cells. However, at the capillary level, the vascular wall consists of a single layer of flattened endothelial cells with a very thin basement membrane and intermittent pericytes without smooth muscle cells [10]. The diameter of perforating arterioles varies by its function and location, and can range from 10 to 240 μm, with the largest one penetrating to the bottom of the sulci [11].
LMC channels consist of small precapillary arterial conduits of varying sizes lying between the pial arteries and arterioles in the cerebral cortex. There are two types of precapillary links: a larger one that connects the surface pial arteries in an end-to-end manner, and a smaller one that bridges smaller perforating arterioles often near the point of cortical penetration. The diameter of these anastomoses shows a wide range (10 to 30 μm) in their resting state [11]. These anastomotic channels work as the anatomical substrate of LMCs beyond an LVO.
Physiology
Perfusion through the arterial/arteriolar anastomoses described above is regulated by CA. CA is a normal physiological response that maintains constant blood flow in response to external and internal stimuli, such as changes in arterial BP between a mean arterial pressure (MAP) of 50 to 170 mm Hg [12,13]. CA provides adequate regional CBF in settings of decreased systemic perfusion or increased local metabolic demand after neuronal/cellular hyperactivity. CA is regulated by a complex interplay of myogenic, neurogenic, metabolic, and endothelial mechanisms [14], all of which modulate the diameter of cerebral vessels. Vascular smooth muscle cells at the precapillary level regulate the vascular diameter through nitric oxide from endothelial cells, neurogenic substances, and cellular metabolic by-products [15-17]. At the capillary level where smooth muscle cells are not found, the pericyte at the arteriolar side may contribute to vessel diameter modulation [18].
As CA depends on the regulation of arteriolar diameters, the functional capacity of CA is determined by the structural and functional properties of the pial artery and arteriolar anastomoses. The normal physiological response of vasodilation to decreased perfusion pressure is altered in various pathological conditions such as aging, long-standing hypertension, and hyperglycemia [19-21]. This resulting impairment in the CA response is not an all-or-none phenomenon but rather a graded and variable response (Figure 1) [22,23].
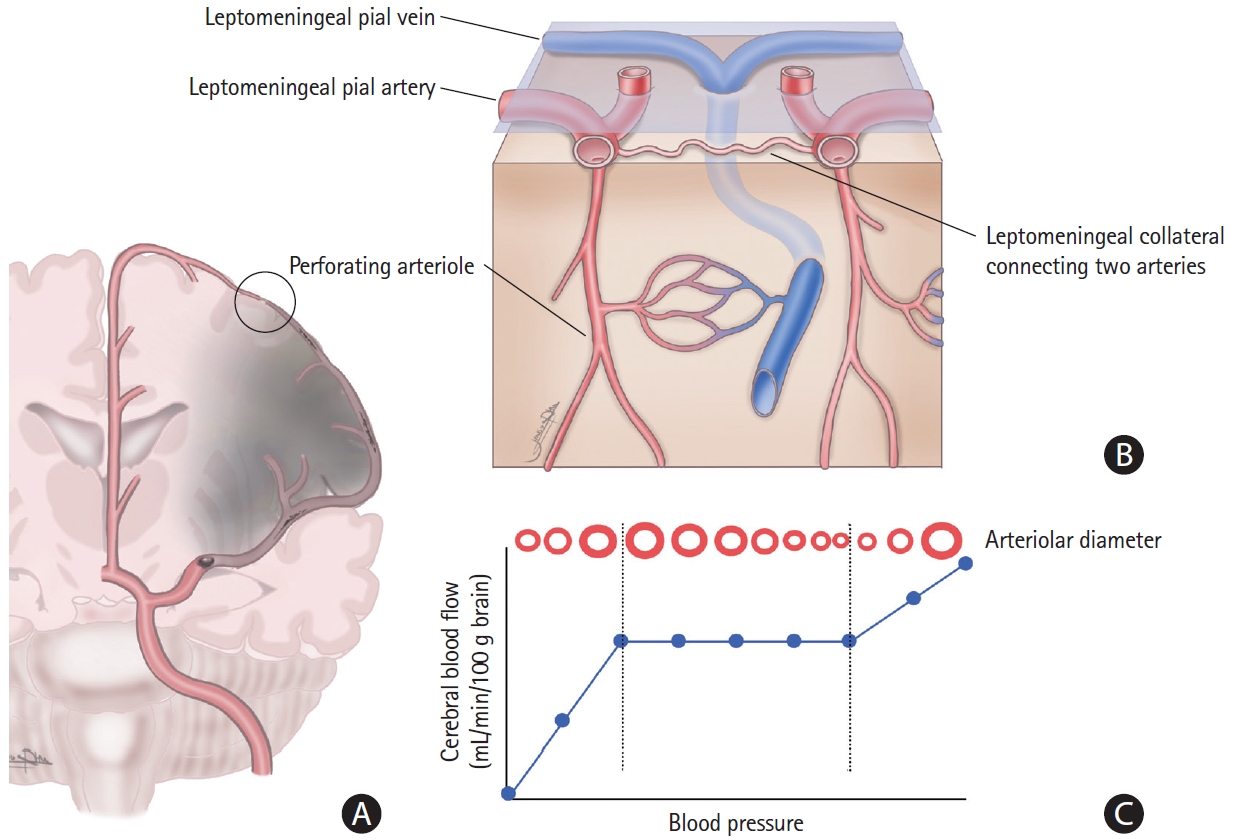
Overview of leptomeningeal collaterals and cerebral autoregulation. (A) When a perfusion pressure gradient develops after large vessel occlusion, leptomeningeal collaterals are instantaneously recruited to provide cerebral perfusion to the ischemic territory within the functional capacity of cerebral autoregulation. (B) Microscopically, leptomeningeal collateral channels utilize pre-existing arterial tubular structures between the pial arteries, arterioles, or proximal branches. (C) The recruitment of leptomeningeal channels depends primarily on the myogenic dilatation of the pial arteries responsive to decreased local perfusion pressure (i.e., cerebral autoregulation).
Leptomeningeal collateral perfusion and systemic blood pressure after large vessel occlusion
After LVO, a significant drop in local perfusion pressure occurs within the affected vascular territory, resulting in a mismatch between metabolic demand and oxygen/nutritional supply. In response to this, LMCs are recruited by vasodilation of the pial arteries and arteriolar anastomoses to their maximum available capacity. The average diameter of these anastomoses is less than 10 to 20 μm in the resting state, but when fully recruited after LVO, their diameter increases almost 6-fold (60 to 120 μm) [24]. Experimental animal model data have shown that this recruitment occurs almost instantaneously after LVO and takes an average of 12 seconds to reach a maximum [25]. As the direction of blood flow through the LMC channel is determined by the perfusion pressure gradient at both ends of the conduit [26], the collateral perfusion passively flows from the ACA or PCA to the ischemic territory in the case of MCA occlusion.
Before successful recanalization is achieved after LVO, the objective of medical management is to prevent irreversible infarction of the ischemic penumbra. Cerebral autoregulatory mechanisms are unable to provide adequate regional CBF due to the severely depressed level of perfusion pressure [27], with the cardiac output now becoming the sole driving force of regional CBF. Informed by this physiological model, stroke physicians do not intentionally lower BP in acute ischemic stroke patients unless it is extremely high [28,29]. However, this simple physiological model does not fully consider the complex interplay between any remaining CA capacity, extent and amount of LMC perfusion, and systemic BP [30]. In this sense, early infarct growth will be a function of time from onset, ischemic severity, that is, baseline LMC perfusion, and tissue vulnerability [31,32].
After successful recanalization, the objective of BP management should be to mitigate the risk of hemorrhagic transformation. The prevailing belief is that lowering BP may prevent hemorrhagic transformation after EVT. However, this simple model does not consider the fact that angiographic recanalization does not always guarantee tissue reperfusion. Examples of such physiology include the “no-reflow” phenomenon and partial recanalization [33,34], situations in which patients may benefit from relatively higher local perfusion pressure [35].
Levels and variabilities of blood pressure in peri-endovascular treatment and stroke outcomes
In the early EVT era, studies mainly focused on one parameter for BP management: BP at the time of hospital arrival (Table 1) [36-42]. Both extremely high and low values of BP on arrival were associated with poor functional recovery or hemorrhagic transformation after EVT [36-38]. The largest dataset to date on this topic was from the Multicenter Randomized Clinical Trial of Endovascular Treatment of Acute Ischemic Stroke in the Netherlands (MR CLEAN) registry with 3,180 EVT cases, which showed a J-shaped association between BP on admission and mortality or functional recovery and an inflection point at 150/81 mm Hg [39]. However, BP at presentation is not modifiable.
The effect of procedural BP during EVT is mostly analyzed in conjunction with the type of anesthesia; therefore, the BP profile in such reports cannot be entirely distinguished from its influence (Table 2) [40,43-61]. It is not uncommon during general anesthesia (GA) to have hypotensive episodes due to anesthesia drugs, intravascular hypovolemia, high intrathoracic pressures, and impaired sympathetic tone [62]. GA is usually correlated with lower BP levels and unfavorable outcomes compared to local anesthesia or conscious sedation [43,44]. The Sedation vs. Intubation for Endovascular Stroke Treatment (SIESTA) and General or Local Anesthesia in Intra-arterial Therapy (GOLIATH) trials were conducted to investigate the association between anesthesia and EVT outcomes [63,64]. Post hoc analyses of these trials reported that stroke outcomes were not associated with the magnitude of BP reduction and type of anesthesia [40,45]. However, subsequent analyses showed that the degree of systolic blood pressure (SBP) or MAP reduction correlated with the volume of infarction and with clinical deficits [46-48]. When individual patient data were combined, longer cumulative durations of MAP less than 70 mm Hg or greater than 90 mm Hg were related to poor functional outcomes [49]. Likewise, data from the MR CLEAN registry showed that a combination of prolonged duration of BP reduction with lower BP level was associated with worse 3-month outcomes [50].
BP measurements obtained after EVT received more attention only after the effectiveness of EVT was proven in clinical trials (Table 3) [40,65-95]. Studies have shown that higher BP after EVT correlates with a higher risk of hemorrhagic transformation, poor functional recovery, and mortality [65-69,96]. The Blood Pressure after Endovascular Therapy for Ischemic Stroke (BEST) study, a multicenter study of 485 EVT cases collected from 12 comprehensive stroke centers, documented that peak SBP <158 mm Hg 24 hours post-EVT was associated with an increased probability of good functional outcomes [70].
Due to the relatively regular interval of BP measurements during the post-EVT phase, the effect of BP variability post-EVT has also been investigated well, with multiple studies showing that higher BP variability is associated with larger infarct volume and poor functional recovery [71,97]. In a secondary analysis of the BEST study, it was shown that the correlation between BP levels and poor functional outcomes became more robust in the group with high BP variability, which suggests an additive effect of BP variability over absolute BP levels [72]. One shortcoming of such analyses is the neglect of the time gap between BP measurements when measuring BP variability. To address this issue, the concept of the rate of BP change, that is, mm Hg/min, was proposed. This rate of change in BP was associated with an increased risk of symptomatic hemorrhage after EVT in one study [73]. Spontaneous reduction of BP after recanalization is well known [98]. In the post-EVT phase, some studies have noted poor outcomes with less BP reduction in those who had relatively higher BP compared to the baseline [70,74]. Current analyses of BP variability, however, continue to be limited. Future analyses may need to incorporate longer-term trajectories and the rapidity of changes in BP readings during the hyperacute period.
Summary of current evidence for clinical practice
Current clinical guidelines and summary of the literature on BP management during the peri-endovascular treatment period
The BP management recommendations from current guidelines may be summarized as follows [28,29]:
(1) Routine use of BP-lowering medication before recanalization treatment (thrombolysis and/or EVT) is not well established and is not recommended unless >220/110 mm Hg.
(2) For IVT alone, it is recommended to maintain a BP <185/110 mm Hg before, during, and 24 hours after treatment.
(3) For EVT, intensive BP lowering in the peri-EVT period lacks clear benefits. It is suggested that BP be maintained at <185/105 mm Hg during and after the procedure. This recommendation was based on recent clinical trial protocols.
The landscape review from the previous section can be summarized as follows:
(1) Admission BP has a J- or U-shaped association with clinical outcomes after EVT.
(2) Prolonged duration of low BP during EVT procedures is associated with unfavorable outcomes.
(3) BP variability after EVT correlates with a higher risk of poor functional recovery and/or increased rates of hemorrhagic complications.
(4) Relatively lower BP reductions after EVT are associated with poor functional recovery.
A tentative suggestion for the BP management of patients with EVT
Pre-EVT period
The objective of BP management during this period should be to maximize the viability of the ischemic penumbra. The recent literature is inconclusive, with both higher or lower levels of admission BPs reported to be correlated with poor outcomes. Current guidelines recommend that EVT candidates maintain an SBP <185 mm Hg. This BP threshold is a legacy from the eligibility criteria for the intravenous thrombolysis trial [99]. Although this threshold BP level was not validated in any of the EVT trials, there is currently no evidence to suggest any other threshold level in these patients. Likewise, there is still minimal evidence to support increased cerebral perfusion after an LVO stroke after iatrogenically induced hypertension. Thus, it is advisable to maintain the BP level that the patient presents before the initiation of EVT.
Procedural BP
Most studies investigating procedural BP levels reported a higher likelihood of poor outcomes after a prolonged duration of lower or higher BP. However, these two points require further discussion. First, these were post hoc analyses of studies that compared the route of anesthesia. Thus, the deleterious effect of low BP cannot be separated from that of anesthetics [48-50]. Second, collateral perfusion through the LMC mainly works before recanalization. The collateral perfusion will instantaneously reverse back to physiological status as soon as the orthotropic flow is restored [5]. Therefore, the procedural BP management strategy may need to distinguish whether recanalization is achieved.
Before recanalization, as in the pre-EVT period, the objective of BP management should be to ensure the viability of the ischemic penumbra. Prolonged exposure to low or high BP should be avoided. A mean arterial BP range between 70 and 90 mm Hg was suggested by one study [49]. After recanalization, the BP management strategy would follow that for the post-recanalization period.
Post-recanalization period
The primary goal of BP management after successful recanalization is to mitigate the risk of hemorrhagic complications and stabilize ischemic tissues. Randomized clinical trials to test the efficacy of EVT for anterior circulation LVO have specified some BP management instructions. The Evaluation Study of Congestive Heart Failure and Pulmonary Artery Catheterization Effectiveness (ESCAPE) trial recommended SBP values ≥150 mm Hg while the artery remains occluded; controlling BP once reperfusion has been achieved while aiming for a normal BP in an individual is sensible [100]. A recent EVT trial proposed SBP <140 mm Hg after successful EVT [101]. In general, higher BP levels during the acute period tend to stabilize after recanalization treatment [98]. Post hoc analyses of the BEST study and the Safe Implementation of Thrombolysis in Stroke International Stroke Thrombolysis Registry (SITS-ISTR) identified that higher BP after EVT is associated with poor outcomes [72,74]. Further studies and discussion should be conducted to investigate whether the unfavorable effect of higher-than-usual BP is reproduced consistently over the recanalization grades, that is, cases with post-EVT recanalization grades modified treatment in cerebral ischemia (mTICI) 2b, 2c, and 3. A patient who had mTICI of 2b recanalization but with occlusion of a distal branch supplying the precentral gyrus may benefit from higher BP levels to support local LMC perfusion. Additionally, the association between BP variability and poor treatment outcomes has been consistently reported in the post-EVT period. Lastly, there is limited evidence that higher BP levels after recanalization cause hemorrhagic transformation.
Thus, the following rule of thumb may be considered until more evidence emerges.
(1) In the post-recanalization period, it is best to maintain the BP level of the patient.
(2) The practice of lowering SBP to less than 140 mm Hg after successful recanalization is not based on proven evidence.
(3) We suggest monitoring BP levels after recanalization and treating only if BP levels are very high; for example, >185 mm Hg systolic when IVT has already been offered or when recanalization grade mTICI 2c or 3 has been achieved.
(4) Higher BP levels after recanalization may portend unfavorable treatment outcomes.
Research perspective
Despite a large body of literature, current clinical guidelines provide limited recommendations regarding BP management strategies in the peri-EVT period. One obvious reason is the retrospective and observational design of published studies. However, BP can be easily measured and readily controlled by parenteral medications. Owing to constant fluctuation in BP during this period, clinical inertia, that is, ignoring outlier BP values, may creep unintentionally. The discrepancy between a prespecified BP target and achieved BP levels is not infrequent in real-world clinical practice. In a recent clinical trial, the BP-Blood Pressure target in Acute Stroke to Reduce Hemorrhage after Endovascular Therapy (TARGET) study randomized 324 cases into an intensive SBP target (100 to 129 mm Hg) and a standard SBP target (130 to 185 mm Hg) during the 24 hours after EVT. The two groups were comparable in radiographic intraparenchymal hemorrhage at 24 to 36 hours, as well as functional recovery and safety outcomes. However, there was an unintended crossover between the assigned target group; patients in the intensive arm spent approximately a third of the duration in the SBP target range of the control arm [75]. Such crossover was also noted in a prospective cohort from eight comprehensive stroke centers that grouped EVT patients into intensive (<140 mm Hg), moderate (<160 mm Hg), and usual (<180 mm Hg) SBP management groups [76]. Despite significant benefits from the moderate target compared to the guideline-recommended target group, the mean SBP levels were almost identical in both groups. Studies focusing on prespecified SBP targeting after EVT need to consider the stabilizing trends of BP after treatment and the inadvertent effect of treating physicians’ behavior and preconceptions.
Summary measures for BP
Repeated measures of BP over time were obtained from a single patient. However, in clinical practice, these measurements are recorded over various time intervals, thus providing only a screenshot of the dynamic readings in time. Considering that every cardiac beat generates SBP and diastolic BP (Figure 2A), the recorded BP values represent only a fraction of the potentially available BP data. Therefore, to capture maximum information, researchers need to carefully choose indices such as BP level, BP variability, and BP trajectory (Figure 2).
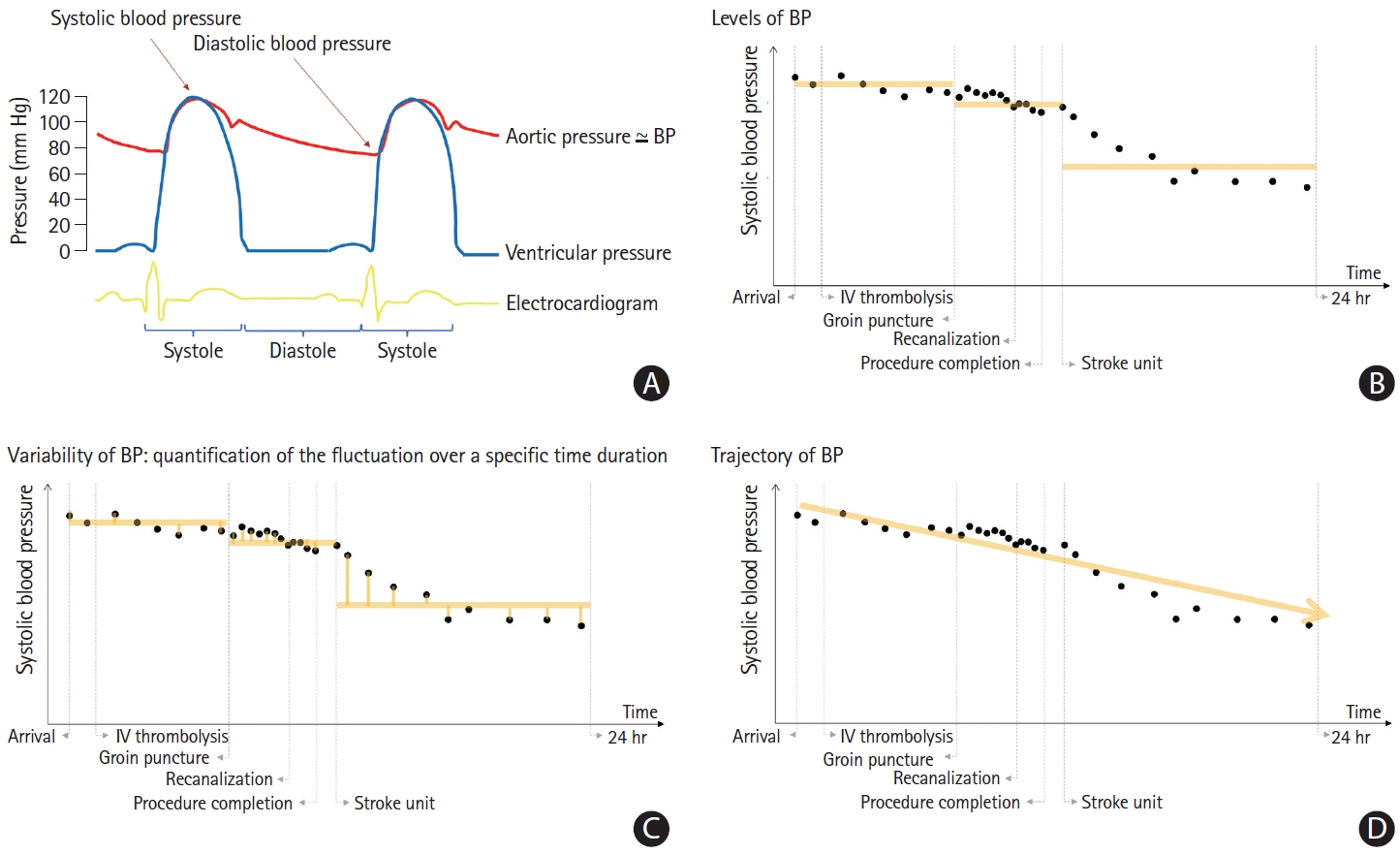
Characteristics of blood pressure measurements and summary indices. Systolic and diastolic blood pressure (BP) is determined during every cardiac cycle. Intermittent measurements in routine clinical practice may capture only a fraction of the available BP measurements. BP measurements can be obtained repeatedly and exhibit constant fluctuations (A). The measurement density of BPs may be different throughout acute in-hospital care, that is, pre-endovascular treatment (EVT), during the procedure, as well as post-EVT. BP measurements can be summarized using means, as highlighted by the yellow line in the figure. The average BP level is intuitive and easy to calculate, but it does not reflect the variability and fluctuations in BP measurements that occur in a patient (B). Fluctuations of BP can be described by various variability measures, including standard deviations, coefficient of variations, maximum decrements, and average real variability, to name a few. In general, variability measures are calculated by taking the differences between each measurement or the differences between specific values (yellow lines). However, such variability measures do not provide information on the time intervals of the measurements. Thus, the absolute BP variability values may decrease during high measurement density periods such as during EVT procedures (C). The trajectory group describes the overall path (yellow line) of BP measurements over a certain period of time. By using a mixture model, clusters of patients with similar patterns of BP measurements over a period of time may be identified and grouped [104]. Five groups of acute BP trajectories were identified from a clinical registry of 8,000 stroke cases [103]. Trajectory groups are easy to recognize in clinical practice even before the measurement period is completed. However, the trajectory group estimation process depends on the characteristics of the source data and the modeling specification, and thus, the generalizability of the model is limited (D). IV, intravenous.
BP level, usually presented as mean BP over a specific duration, expresses the status of BP at a particular time point or period. This level is intuitive to physicians, and the change in the BP level may provide practical information (Figure 2B) [74]. However, this measure does not capture information about the BP variation generated by every cardiac beat. Variability is a summary measure of the fluctuation of the measurements over a specific duration (Figure 2C). It is not easy to set up a specific threshold of BP variability that warrants clinical intervention, as accurately measuring variability requires a sufficient count of BP measurements to be calculated. It should also be acknowledged that variability indices are affected by the temporal density of BP measurements and BP levels. The absolute BP variability value may decrease during a high measurement density period, that is, during the EVT procedure. Short-term variability is also affected by physical activity, emotional stress, and circadian rhythm, all of which augment the complexity of interpretation of such data [102]. BP trajectories focus on identifying a specific group following a similar trend and pattern of the BP measurements over a certain period (Figure 2D) [103,104]. Trajectory grouping and estimation procedures currently require specific model specifications and are not widely applied to the acute stroke period.
The relationship between leptomeningeal collaterals and blood pressure in patients with large vessel occlusions
As discussed in this review, regional tissue perfusion after LVO is maintained by the LMC channels. The recruitment of these LMC channels is determined by the pressure gradient between the arterial blood flow generated by cardiac contraction and venous blood flow. Based on this simple and linear model, it is intuitive to suppose that cerebral tissue perfusion after LVO may be regulated through systemic BP management.
However, the small arteries and arterioles of the brain have CA capacity, which modulates the vascular diameter to maintain almost constant CBF in response to local perfusion pressure changes. Therefore, the brain tissue that may receive increased local CBF from augmenting systemic BP is likely to be either the ischemic core or the ischemic penumbra without autoregulatory function. A long history of hypertension may indicate impaired autoregulatory function [105]. Additionally, as the blood flow inversely corresponds to the fourth-order of the vascular luminal diameter, the LMC perfusion will not suffice to replace the normal physiologic CBF through anatomically intact large vessels.
Based on the above, the following can be stated:
(1) The effect of systemic BP on blood flow distal to an LVO may vary based on the anatomic extent of the LMC channels and the remaining autoregulatory function.
(2) Ischemic brain injury may affect the vascular endothelium, pericytes, glia, and neuronal cells, and it prevents the maintenance of normal autoregulatory function. The autoregulatory function may also be affected by prior conditions, such as hypertension and diabetes mellitus.
(3) In general, LMC perfusion only provides inadequate blood flow that cannot sustain brain tissue viability for long.
(4) The BP management strategy for acute ischemic stroke patients may need to be personalized based on the individual assessment of the LMC channels and autoregulatory capacity.
Whether baseline hemodynamic status modifies the association between BP profiles and ischemic or functional outcomes is a fascinating research topic [106]. This issue has recently gained interest with studies showing that poor baseline collaterals can aggravate the deleterious effects of higher BP levels or higher BP variability on clinical outcomes [51,77]. The association between BP and stroke outcomes may also differ by baseline perfusion status, that is, the extent and grade of LMC and tissue perfusion. As discussed in the previous section, early infarct growth may be a function of time from onset, ischemic severity (i.e., baseline LMC perfusion), and tissue vulnerability [31,32]. It can be speculated that in patients with poor LMCs after LVO, ischemic injury rapidly develops, and the only effective treatment strategy is to expedite recanalization. In contrast, in the presence of moderate LMCs, tissue viability mainly depends on systemic perfusion, and clinicians should be sensitive to even minute changes in BP. Meanwhile, in patients with excellent or good collaterals, one can expect a higher tolerance to modest BP fluctuations. This conjecture needs to be validated in future studies.
Conclusions
In current practice, stroke physicians use BP measurements as a proxy for brain perfusion pressure. Conceptually, the objective of BP management in the pre-recanalization phase is to maintain sufficient LMC perfusion. In the post-recanalization phase, the objective is to prevent hemorrhagic complications and stabilize the ischemic brain tissues. At present, the available research on BP management in EVT shows that higher BP on admission, lower BP levels post-recanalization, higher BP variability or variable trajectories, and prolonged durations of low BP during EVT are all associated with poor functional outcomes. However, the optimal BP level, variability, and trajectory for each phase are yet to be determined. Based on current anatomical and physiological knowledge, the ultimate goal is to directly gauge the amount and capacity of LMC perfusion after LVO to provide maximally available CBF to the ischemic brain until successful recanalization is achieved and to mitigate undesirable complications subsequent to ischemia and reperfusion. Given the heterogeneity of the anatomy and variability of the physiology of the LMC, understanding its behavior during stroke is now more pressing than ever. It is paramount that more novel mechanisms and parameters to assess regional brain perfusion and functioning CA capacity at the bedside are developed in order to individualize BP management in the periEVT period [52,78].
Notes
Disclosure
The authors have no financial conflicts of interest.