A Practical Grading Scale for Predicting Outcomes of Radiosurgery for Dural Arteriovenous Fistulas: JLGK 1802 Study
Article information
Abstract
Background and Purpose
To assess the long-term outcomes of intracranial dural arteriovenous fistula (DAVF) treated with stereotactic radiosurgery (SRS) alone or embolization and SRS (Emb-SRS) and to develop a grading system for predicting DAVF obliteration.
Methods
This multi-institutional retrospective study included 200 patients with DAVF treated with SRS or Emb-SRS. We investigated the long-term obliteration rate and obliteration-associated factors. We developed a new grading system to estimate the obliteration rate. Additionally, we compared the outcomes of SRS and Emb-SRS by using propensity score matching.
Results
The 3- and 4-year obliteration rates were 66.3% and 78.8%, respectively. The post-SRS hemorrhage rate was 2%. In the matched cohort, the SRS and Emb-SRS groups did not differ in the rates of obliteration (P=0.54) or post-SRS hemorrhage (P=0.50). In multivariable analysis, DAVF location and cortical venous reflux (CVR) were independently associated with obliteration. The new grading system assigned 2, 1, and 0 points to DAVFs in the anterior skull base or middle fossa, DAVFs with CVR or DAVFs in the superior sagittal sinus or tentorium, and DAVFs without these factors, respectively. Using the total points, patients were stratified into the highest (0 points), intermediate (1 point), or lowest (≥2 points) obliteration rate groups that exhibited 4-year obliteration rates of 94.4%, 71.3%, and 60.4%, respectively (P<0.01).
Conclusions
SRS-based therapy achieved DAVF obliteration in more than three-quarters of the patients at 4 years of age. Our grading system can stratify the obliteration rate and may guide physicians in treatment selection.
Introduction
Intracranial dural arteriovenous fistula (DAVF) is an acquired arteriovenous shunt between the dural arteries and cortical veins or dural sinuses [1,2]. Since Fincher’s initial report in 1951 [3], DAVF cases have continuously been documented. DAVFs are now recognized as one of the main forms of central nervous system vascular malformations. The associated symptoms include bruit and venous impairments secondary to intracranial venous occlusion, stasis, and reflux. These abnormalities include intracranial hemorrhage, ocular symptoms, and non-hemorrhagic neurological deficits (NHNDs) [1,2]. As suggested by commonly used grading systems, such as those of Borden et al. [4] and Cognard et al. [5], the risk posed by DAVF is mainly dependent on the presence of cortical venous reflux (CVR [6,7]). DAVFs without CVR rarely cause hemorrhage or NHND. DAVFs with CVR have an annual risk of hemorrhage of up to 7.6% [8]. Nevertheless, low-risk DAVFs may also develop similar aggressive features over time [9,10].
Stereotactic radiosurgery (SRS), which involves the delivery of a precise, high-dose of radiotherapy in a single session, is known to be effective for treating arteriovenous shunt disorders [11]. The relevant evidence has been obtained mainly from studies on arteriovenous malformations (AVMs). However, there remains a paucity of high-quality evidence from largescale investigations of patients with DAVFs. Accordingly, many neurosurgeons consider endovascular embolization and direct surgery as the current standard therapeutic modalities for DAVFs. SRS is generally considered an alternative when endovascular embolization and/or direct surgery fail or are not feasible. Nevertheless, a gradual but steady accumulation of retrospective evidence suggests that SRS has a similar level of efficacy to other modalities. In addition, SRS has the added advantage of minimal invasiveness.
Several issues must be clarified before establishing SRS as an effective treatment option for DAVFs. First, the factors associated with favorable outcomes must be clarified. The creation of a practical radiosurgical grading scale would be invaluable for guiding the optimal application of SRS. Second, many patients with DAVF undergo SRS with embolization (Emb-SRS). Evaluating SRS outcomes as a standalone treatment and comparing them to Emb-SRS treatment is essential to develop a better understand of the role of SRS as a standalone modality. To accomplish these goals, a study that includes many patients is required for appropriate statistical analyses. However, such studies are challenging to perform at a single institution, given the rarity of SRS cases. The present nationwide, multi-institutional study was conducted in collaboration with the Japanese Leksell Gamma Knife Society.
Methods
Data collection
The data of 253 patients with DAVFs who were treated between 1997 and 2016 at 13 Japanese gamma knife centers affiliated with the Japanese Leksell Gamma Knife Society were retrospectively collected. Complete enumeration was mandated to minimize reporting bias aside from the following exclusion criteria: (1) history of extensive intracranial radiotherapy (except for stereotactic radiotherapy for lesions far from the DAVF); (2) no radiographic follow-up; and (3) age below 20 years at SRS. After data merging, 40 patients with multiple DAVFs, 12 who had undergone direct surgery, and one for whom sufficient data were not obtained were further excluded. Thus, a total of 200 patients were included in the study. An itemized list of the extracted information is shown in Supplementary Table 1. NHND was defined as any progressive focal neurological deficit with features other than headache, bruit, orbital phenomena, and cranial nerve deficits due to cavernous sinus (CS) lesions (ophthalmoplegia), which are usually regarded as benign [12,13].
The therapeutic strategy for DAVF was determined by each gamma knife center, referring hospitals, or a combination of these. This determination was made after due consideration of the location, symptoms, neurological deficits, presence/absence of hemorrhage, and CVR, as well as the patient’s preference. In general, endovascular treatment was primarily selected for those who had hemorrhagic strokes and/or impending neurological symptoms. SRS tended to be selected for those who did not have impending neurological symptoms. Surgical treatment was generally reserved for specific situations, such as anterior skull base (ASB) DAVFs.
All aspects of this study were approved by the Clinical Research Review Board of our institution (2018187NI). The study was performed in compliance with the institutional and Strengthening The Reporting of OBservational Studies in Epidemiology (STROBE) guidelines (Appendix 1). This study was registered in a clinical trial registry (University Hospital Medical Information Network Clinical Trials Registry, UMIN000037211) and certified by the Academic Committee of the Japanese Leksell Gamma Knife Society (JLGK1802). The requirement for patient consent was waived owing to the retrospective nature of the study.
Radiosurgical techniques and post-radiosurgical follow-up
A Leksell Gamma Knife (Elekta AB, Stockholm, Sweden) was used for SRS at all institutions. The Gamma Knife model (B, C, 4C, and Perfexion) and treatment software (KULA and GammaPlan, Elekta AB) were selected based on their availability at each institution at the time of SRS. Each patient underwent placement of a stereotactic Leksell frame (Elekta AB) under local anesthesia with or without mild sedation. For stereotactic imaging, a combination of magnetic resonance imaging and digital subtraction angiography (DSA) was generally preferred to computed tomography angiography. However, the technique to be used was determined at the discretion of the attending physicians, and the choice was dependent on the availability of equipment at each center. During radiosurgical planning, arteriovenous fistulas in the dural tissues, appearing as a conglomeration of abnormal small vessels, were circumscribed. Dose selection was performed at the discretion of each center. A prescription dose of 18 to 20 Gy was usually preferred. Because the target location was outside the brain tissues, dose reduction was rarely required. The radiosurgical target tended to be slightly more generous than that for intra-axial lesions.
Patients were followed up regularly at each gamma knife center or referring hospital. In general, magnetic resonance imagings were performed every 6 months until fistula obliteration was confirmed and annually thereafter. DSA was mainly used to confirm fistula obliteration. However, magnetic resonance imaging and/or computed tomography angiography were used alternatively if the patient declined DSA.
Statistical analysis
First, the rate of fistula obliteration was analyzed using the Kaplan-Meier method. The curves were stratified based on the baseline characteristics. Each pair of curves was compared using the log-rank test. Continuous variables were dichotomized using median values and were used for stratification. Given the multiplicity, the locations of the fistulas were grouped based on the odds ratios. Patients who underwent any secondary intervention for DAVF were censored at that point (censoring without obliteration). Factors associated with obliteration were examined using bivariate and multivariate Cox proportional hazards analyses. Based on bivariate analysis, factors with a P<0.10 were entered into the multivariate model. Post-SRS hemorrhage was examined using the person-year method. Adverse radiation events (AREs) were summarized and graded using the Common Terminology Criteria for Adverse Events (CTCAE v5.0). Disease-specific mortality was determined using the Kaplan-Meier method. Neurological status prior to SRS and at the last clinical visit was retrieved from patient medical records. Subsequently, the modified Rankin Scale (mRS) [14] score was retrospectively assigned.
Next, to examine the effect of embolization, radiosurgical outcomes were compared between patients treated with SRS alone and those treated with Emb-SRS. The 1:1 nearest available matching method was used. Matching variables were determined based on differences in baseline characteristics between the two cohorts. The rates of obliteration and post-SRS hemorrhage were compared between the matched cohorts.
Finally, to develop a practical grading scale for the prediction of fistula obliteration, factors predictive of fistula obliteration in multivariate analysis were weighted according to their odds ratios. The area under the receiver operating characteristic curve was generated to evaluate the predictive accuracy of the model. Although there is no radiosurgical grading scale for DAVFs, the Virginia Radiosurgery AVM Scale is widely used [15]. The goodness-of-fit of this scale was assessed using the R2 value (0.090). Similarly, we also calculated the R2 value for the supplemental evaluation of the goodness-of-fit of our scale. All statistical analyses were performed using JMP Pro version 15.0 (SAS Institute Inc., Cary, NC, USA). Statistical significance was set at P<0.05.
Results
Baseline characteristics and overall radiosurgical outcomes
The baseline characteristics are summarized in Table 1. The median radiological follow-up period was 44.6 months (interquartile range [IQR], 24.2 to 73.0). A total of 156, 123, and 92 patients completed the imaging evaluation at 2-, 3-, and 4-year follow-up, respectively. Fistula obliteration was confirmed in 142 (71.0%) patients at a median of 19.9 months (IQR, 12.0 to 28.0); DSA was used in 116 (81.7%) of these patients. The 2-, 3-, 4-, and 5-year cumulative obliteration rates were 49.6%, 66.3%, 78.8%, and 82.7%, respectively (Figure 1A). For residual DAVFs, additional interventions were performed in 15 patients at a median of 38 months (IQR, 12 to 50). Additional interventions included embolization, SRS, direct surgery, and a combination of SRS and embolization in six, five, three, and one patients, respectively. Further data on fistula obliteration following additional interventions were not consistently available.

Patients’ baseline characteristics: summary and comparisons between patients treated with SRS alone and Emb-SRS

Kaplan-Meier curves of fistula obliteration rates. (A) Entire cohort, (B) female vs. male, (C) presence vs. absence of cortical venous reflux (CVR), (D) sinus type vs. non-sinus type, (E) per fistula location, (F) per location category. SRS, stereotactic radiosurgery; CS, cavernous sinus; TS, transverse-sigmoid; SSS, superior sagittal sinus; ASB, anterior skull base; MF, middle fossa; Tent, tentorial.
The obliteration rate significantly differed according to sex, drainage pattern, sinus versus non-sinus DAVF type, and fistula location. The obliteration rate was higher in women versus men (P<0.01), DAVFs with versus without CVR (P<0.01), and sinus type versus non-sinus type (P<0.01). DAVFs in the CS, transverse-sigmoid (TS) sinus, or other locations had the highest obliteration rates, followed by those in the superior sagittal sinus (SSS), tentorium (Tent), ASB, and middle fossa (MF) (P<0.01) (Figure 1B-E). Other factors such as age (<65 years vs. ≥65 years), prior embolization, hemorrhagic onset, presence of NHND, prescription dose (<20 Gy vs. ≥20 Gy), central dose (<36 Gy vs. ≥36 Gy), and target volume (≤1.5 mL vs. >1.5 mL) failed to show significant differences. Based on the odds ratios for obliteration, fistula locations were categorized into three groups: (1) ASB/MF (lowest expectation of obliteration); (2) SSS/Tent (medium); and (3) CS/TS/others (highest). Significant differences in the obliteration outcomes were observed across the three location categories (P<0.01) (Figure 1F).
Based on the univariate Cox proportional hazard analysis, sex, location category, CVR, and sinus/non-sinus type were entered into the multivariate model, which demonstrated that location category (adjusted hazard ratio and 95% confidence interval [CI] for CS/TS/others [vs. ASB/MF], 4.0 [95% CI, 1.5 to 10.4]; CS/TS/others [vs. SSS/Tent], 1.8 [95% CI, 1.1 to 3.0]; and SSS/Tent [vs. ASB/MF], 2.2 [95% CI, 0.8 to 5.8]; P-value for overall difference <0.01) and absence of CVR (adjusted hazard ratio, 1.7; 95% CI, 1.2 to 2.5; P<0.01) were significantly associated with obliteration (Table 2).

The results of bi- and multi-variate Cox proportional hazard analyses for factors potentially associated with obliteration
Post-SRS hemorrhage occurred in four patients (2.0%) at a median of 9.3 months (IQR, 5.0 to 61.6). The details are summarized in Supplementary Table 2. All hemorrhages were caused by unobliterated niduses. Three patients (75.0%) presented with hemorrhage or NHND and two (50%) had CVR. Mild/temporary deficits were observed in two patients, whereas no deficits occurred in the remaining patients. Three hemorrhages occurred over 369.3 person-years within 2 years following SRS, and one hemorrhage occurred over 617.5 person-years thereafter. Thus, the annual hemorrhage rates were 0.8% for the first 2 years and 0.5% thereafter.
Regarding symptom improvement, bruit (n=31) improved in 27 patients (87.1%) at a median of 6.0 months (IQR, 3.0 to 9.0), ophthalmic symptoms (n=47) improved in 35 patients (74.4%) at a median of 5.0 months (IQR, 3.0 to 10.5), NHND (n=24) improved in eight patients (33.3%) at a median of 5.5 months (IQR, 2.3 to 11.1), and AREs were observed in six patients. The AREs included right upper extremity weakness due to a DSA complication in one patient (grade 4), temporary abducens and trigeminal nerve deficits in one patient (grade 2; improved with oral corticosteroid), and asymptomatic edema adjacent to the irradiated field in four patients (grade 1). Thus, the incidence of symptomatic AREs was 1.0%. During follow-up, there were four deaths (three due to extracranial malignancy and one due to a dissecting aortic aneurysm). Therefore, the disease-specific mortality rate was 0%. The preoperative mRS score was 0 in 82 patients (41.0%), 1 in 79 (39.5%), 2 in 23 (11.5%), 3 in 10 (5.0%), and 4–5 in six (3.0%). At the last clinical visit, the mRS score was 0 in 146 patients (73.0%), 1 in 20 (10.0%), 2 in 18 (9.0%), 3 in seven (3.5%), 4–5 in five (2.5%), and 6 in four (2.0%). Thus, the mRS scores improved in 75 patients (37.5%), remained unchanged in 117 (58.5%), and worsened in eight (4.0%). Deterioration in mRS scores was largely unrelated to SRS or DAVF (remote malignancy in four patients, aortic dissection in one, Parkinson’s disease in one, and senility in one), except for one patient who developed ataxia from post-SRS hemorrhage.
Effect of embolization on obliteration and hemorrhage
In total, 83 patients were treated with SRS alone, and 117 underwent Emb-SRS. Regarding Emb-SRS, embolization preceded SRS in all but three patients who received SRS and planned adjuvant embolization within 2 months following SRS. The materials used for embolization included the following: n-butyl-2-cyanoacrylate (n=37), coil (n=26), n-butyl-2-cyanoacrylate with coil (n=18), polyvinyl alcohol/gelatin sponge (n=4), coil with polyvinyl alcohol particles (n=3), n-butyl-2-cyanoacrylate with polyvinyl alcohol particles (n=2), Onyx (Medtronic, Dublin, Ireland; n=1), triacyl gelatin microspheres (Embosphere, Nippon Kayaku, Tokyo, Japan; n=1), absorbable sutures (n=1), and unknown (n=24). The baseline characteristics of the patients are summarized in Table 1. There was no significant difference in the baseline characteristics, except for less frequent NHNDs (P<0.01), additional incidental DAVF detection (P=0.03), and a decreased radiation target volume (P<0.01) in the SRS alone group compared to the Emb-SRS group.
Matching was performed using a 1:1 protocol without replacement, with a caliper width equal to 0.2 of the standard deviation of the logit of propensity. Factors that were significantly different between the cohorts (target volume, onset of NHND, and incidental diagnosis) and significantly associated with obliteration (location category and CVR) were used as matching variables. Thus, two matched cohorts comprising 146 patients were included. There were no significant differences in the baseline characteristics between the cohorts (Supplementary Table 3). Fistula obliteration following initial SRS was confirmed in 51 (69.9%) and 53 (72.6%) patients in the SRS alone and Emb-SRS groups, respectively. Emb-SRS was not associated with fistula obliteration (4-year cumulative obliteration rates [Emb-SRS vs. SRS alone], 80% vs. 76%; P=0.54; hazard ratio, 1.13; 95% CI, 0.78 to 1.66) (Figure 2). Post-SRS hemorrhage occurred in two (2.7%) and no (0%) patients in the SRS alone and Emb-SRS groups, respectively. No significant difference in the incidence of post-SRS hemorrhage was observed between the cohorts (Fisher exact test, P=0.50).
Development of a practical radiosurgical grading system for DAVFs
The results of the analyses described above showed that the location category and CVR were significantly associated with fistula obliteration. To develop a radiosurgical DAVF grading system, these factors were weighed according to the odds ratios for failed obliteration (presence of CVR [vs. absence], 3.0 [95% CI, 1.5 to 5.9; P<0.01]; SSS/Tent [vs. CS/TS/others], 3.3 [95% CI, 1.6 to 6.9; P<0.01]; ASB/MF [vs. CS/TS/others], 11.8 [95% CI, 3.7 to 38.3; P<0.01]). As such, in the grading system, no points were assigned if the patient had a fistula without CVR and that was not located in the ASB, MF, SSS, or Tent; 1 point was assigned if the fistula had CVR or was located in the SSS or Tent; and 2 points were assigned if the fistula was located in the ASB or MF. Using the total points assigned, patients were divided into three groups based on the expectation of fistula obliteration: highest (0 points), intermediate (1 point), and lowest (≥2 points) (Figure 3). According to this grading system, the 2-, 3-, and 4-year cumulative obliteration rates were 74.2%, 85.1%, and 94.4% in the highest expectation group; 42.3%, 62.7%, and 71.3% in the intermediate expectation group; and 14.6%, 34.7%, and 60.4% in the lowest expectation group (P<0.01), respectively. Logistic regression analysis demonstrated that the area under the curve of the model was 0.75, and the R2 value was 0.24, which was comparable to the pseudo R2 value (0.090) achieved by the Virginia Radiosurgery AVM Scale [15].
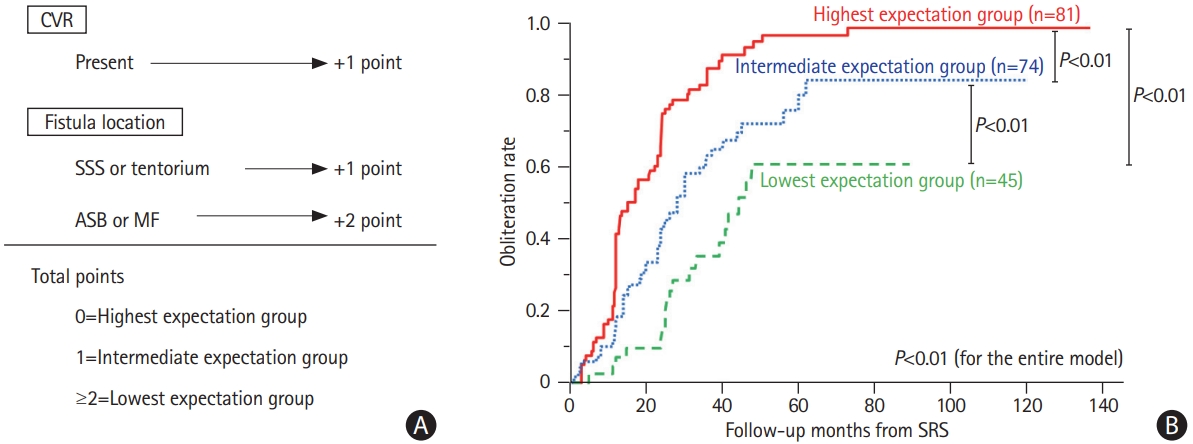
Radiosurgical grading system for dural arteriovenous fistulas (DAVFs). (A) Schematic drawing of the radiosurgical DAVF grading system. (B) Kaplan-Meier curves showing rates of fistula obliteration stratified by the radiosurgical DAVF grading system. CVR, cortical venous reflux; SSS, superior sagittal sinus; ASB, anterior skull base; MF, middle fossa; SRS, stereotactic radiosurgery.
Discussion
In this retrospective, multi-institutional study on SRS for DAVF, the overall outcomes were satisfactory, with a favorable obliteration rate (78.8% at 4 years) and a low symptomatic ARE rate (1.0%). These results are largely in line with those of earlier studies [16-26]. Approximately 75% of post-SRS hemorrhages occurred during the latency phase during the process of fistula obliteration. Although the lack of an immediate effect is a major shortcoming of SRS, the hemorrhage rate (2.0%) was acceptable, and the neurological sequelae were generally mild. Improvements in symptoms, especially bruit and ophthalmic symptoms, were achieved in most patients and occurred earlier than fistula obliteration (median, 5.0 to 6.0 months). The number of patients (n=200) in our study is the largest ever reported, which supports the validity and robustness of our results. The main achievement of this study was the development of a scoring system. This system was based on the location category and the presence/absence of CVR that could successfully predict the likelihood of SRS-induced fistula obliteration. The area under the curve of the system (0.75) indicated a good predictive accuracy [27], and the R2 value (0.24) was comparable to that achieved using the Virginia Radiosurgery AVM Scale (0.090) [15]. No grading system predicting the results of SRS for DAVF has ever been established. Consequently, our scoring system appears to be especially practical and may guide surgeons in determining more appropriate, situation-based therapeutic strategies. The generalizability of this finding requires further evaluation in future studies.
Identifying the factors associated with favorable outcomes is essential for the safe and effective application of SRS. As noted in a previous study, the commonly used grading scales for DAVFs, such as Borden’s and Cognard’s scales, are not useful in this regard [28]. Because of the rarity of both DAVF itself and its treatment with SRS, few previous studies involving relatively large numbers of patients have found factors possibly associated with fistula obliteration. Examples of these factors include absence of CVR [16,19], CS location [16,28], female sex [28], and absence of venous ectasia [28]; however, no factor has been consistently identified. Our results confirmed a significant association between the absence of CVR and obliteration. The reason for this association remains unclear; however, hemodynamic factors may play a significant role, given that the presence of CVR implies intolerably increased pressure in the shunting point/draining sinus. Another factor may be the large diameters of the shunting vessels, which presumably lead to a high-flow shunt and thus an increase in pressure. Regarding fistula location, we not only confirmed the previous finding that CS location was associated with obliteration but also observed that the likelihood of obliteration could be stratified by the location category designated as lowest (ASB and MF), intermediate (SSS and Tent), and highest (CS, TS, and others). DAVFs in CS/TS are in the proximity of the large dural sinuses, while those in the ASB/MF are not. One possible explanation for this association is that an anatomical relationship to a large dural sinus can potentially “buffer” elevated venous pressure. In contrast, although female sex was significantly associated with obliteration in bivariate analysis, an independent association was not observed in the multivariate analysis. The strong female predominance of CS-DAVFs [28-30] seems to explain this ostensible relationship. Indeed, a significant association between female sex and obliteration has not been confirmed in most recent studies [16,19,30-32]. We did not collect information on venous ectasia. Thus, the significance of this factor remains to be elucidated. However, venous ectasia theoretically occurs because of elevated venous pressure in the cortical veins with regurgitation, which seems to have the same pathophysiological significance as CVR. Unlike AVMs, radiosurgical doses or target volumes were not significantly associated with obliteration, similar to previous studies [16,19,30-32]. This result may be attributed to the fact that these factors were more homogeneous than AVM characteristics. Despite our results, prescription doses should be maintained at 18 Gy or higher, given that such doses have been used in many previous studies as well as the present study [16,28,32-36].
A combination of SRS and embolization has been documented in several studies and has become a commonly used treatment strategy for DAVFs [25,26,36]. Although the true effect of SRS on AVMs remains debatable, several studies have suggested that embolization negatively affects SRS outcomes [16,37]. In contrast, embolization can be safely incorporated into SRS for DAVFs. Our comparisons and matched cohort analyses showed no negative effects of embolization. This finding also implies that embolization combined with SRS may not confer a definitive benefit. However, we should interpret these results with caution, as our cohort was likely subject to selection bias. In fact, NHNDs were significantly less common in the SRS-alone group than in the Emb-SRS group. Nevertheless, in the SRS alone group, there were comparable numbers of patients with purported aggressive features such as CVR (38.6% vs. 48.7%), non-sinus-type DAVFs (13.3% vs. 20.0%), and hemorrhagic onset (16.9% vs. 21.4%). The latter of these two factors did not appear to significantly reduce the obliteration rate. Although CVR has been demonstrated to reduce the obliteration rate, fistula obliteration could be achieved in approximately 70% of these patients. Given the satisfactorily low rates of symptomatic AREs and post-SRS hemorrhages, SRS alone appears to be safe and effective and may be worth considering for such patients. However, it should be noted that endovascular embolization and subsequent early obliteration or flow reduction of the arteriovenous shunt may be beneficial for impending cases, such as DAVFs with early rebleeding and progressive focal neurological deficits other than ophthalmoplegia.
This study has a few limitations. First, selection bias was unavoidable given the retrospective nature of the study. Therefore, cautious interpretation of the results of comparisons between SRS alone and Emb-SRS is necessary, as described above. This fact might also impair the external validity of the new scoring system, for which further evaluation is required. Therefore, the generalizability of this study to individual cases should be considered. Moreover, multi-institutional studies tend to be subject to reporting bias. Therefore, to minimize bias, complete enumeration was mandated in this study. Furthermore, fistula obliteration was confirmed based on magnetic resonance imaging/computed tomography angiography findings in 19.3% of patients; thus, a slight overestimation of the obliteration rates might have occurred. Finally, although Emb-SRS exhibited the same level of efficacy as SRS alone, embolization-associated adverse events must be considered.
Conclusions
SRS was found to be effective for DAVFs, with an estimated 4-year cumulative obliteration rate of 78.8%, annual post-SRS hemorrhage rates of 0.8% (≤2 years) and 0.5% (>2 years), and a crude symptomatic ARE rate of 1.0%. In addition to the fact that embolization does not appear to adversely affect SRS, SRS alone may have a similar level of efficacy to Emb-SRS and can be considered feasible, except in impending situations in which endovascular embolization and subsequent early obliteration or flow reduction of the arteriovenous shunt may be beneficial. It remains unclear whether Emb-SRS is superior to SRS alone in certain DAVFs. Multivariate analysis revealed that CVR and location category (ASB/MF vs. SSS/Tent vs. CS/TS/others) were associated with fistula obliteration. The radiosurgical grading system developed using these factors could successfully stratify patients into three groups: highest, intermediate, and lowest expectations of obliteration. These findings may help determine more appropriate and tailored therapeutic strategies for DAVFs.
Supplementary materials
Supplementary materials related to this article can be found online at https://doi.org/10.5853/jos.2021.03594.
List of extracted information
Details of patients who developed post-SRS hemorrhage
Comparison of baseline characteristics between the SRS alone and Emb-SRS groups following propensity score matching
Notes
Disclosure
The authors have no financial conflicts of interest.
Acknowledgements
This study was funded by an Elekta research grant.